What are the underlying processes driving highly effective and efficient thermal management solutions? A novel approach to thermal optimization promises significant advancements.
This specialized thermal technology emphasizes deep penetration and intense heat management. It leverages advanced materials and methodologies to achieve extremely high rates of heat dissipation or absorption. The core principle revolves around the precise control of thermal fluxes at microscopic and macroscopic scales. Examples include innovative cooling systems for high-performance electronics and optimized processes in materials science.
The benefits of such a focused approach are substantial. Significant improvements in performance can be realized through optimized thermal pathways. This translates into enhanced operating efficiency for various applications. Longer lifespan and reliability can also be expected by mitigating the detrimental effects of overheating. This technology allows for the design of more powerful and compact devices. It's pivotal in areas like data centers, microelectronics, and industrial processes. Advanced thermal analysis and modeling are essential components for the continued development and refinement of this methodology.
This examination of high-performance thermal solutions lays the groundwork for a deeper dive into the specific techniques and applications of this transformative technology. Further exploration will detail the material science advancements, computational modeling techniques, and real-world applications underpinning this approach.
Deephot
Understanding the core components of deephot is crucial for appreciating its multifaceted implications. This analysis examines eight key aspects vital to comprehending this innovative approach.
- Thermal management
- Advanced materials
- High efficiency
- Precise control
- Microscopic scale
- Macroscopic scale
- Heat dissipation
- Performance enhancement
These key aspects highlight the integrated nature of deephot. Thermal management, for instance, relies on advanced materials for high efficiency, accomplished through precise control at both microscopic and macroscopic levels. Heat dissipation is optimized, leading to performance enhancement. Deephot's efficacy stems from its ability to simultaneously consider both micro- and macro-scale effects. Applications in microelectronics leverage these principles to reduce overheating, thereby improving device longevity and functionality. Deephot's multi-faceted approach holds promise for groundbreaking advancements in diverse fields, demanding deep exploration to realize its full potential.
1. Thermal Management
Effective thermal management is fundamental to the functioning of numerous systems, particularly those operating at high power densities. Deephot represents a specialized approach to thermal management, emphasizing exceptionally efficient heat dissipation or absorption. This enhanced control over thermal processes is critical in applications requiring high performance and extended lifespan. A crucial aspect of deephot lies in its meticulous attention to heat flow at both microscopic and macroscopic levels. This approach allows for precise targeting and modulation of thermal fluxes, leading to significantly improved performance in systems like advanced microelectronics, where heat generation can quickly lead to performance degradation or failure.
Real-world examples of the significance of thermal management are abundant. High-performance computing systems rely heavily on effective cooling mechanisms to prevent overheating and maintain optimal performance. Similarly, modern microprocessors generate immense heat, requiring sophisticated thermal management solutions to ensure reliability and prevent failures due to thermal stress. The development of advanced materials and innovative designs, epitomized by deephot, directly addresses these challenges. By tailoring thermal pathways, deephot minimizes thermal resistance and enhances heat transfer, leading to significant performance improvements and extended operational lifetimes. The strategic incorporation of deephot principles enables the creation of more powerful and compact devices capable of enduring demanding operational environments.
In conclusion, thermal management is not merely an ancillary function but rather a cornerstone of deephot's innovative approach. The pursuit of optimized thermal pathways and the precise manipulation of heat transfer processes are central to the practical application of deephot. Understanding the intricate relationship between thermal management and the specific techniques employed by deephot is paramount to realizing its full potential in various high-performance sectors. The effective application of this methodology holds the key to enabling the development of even more advanced and efficient systems in the future.
2. Advanced Materials
Advanced materials play a critical role in enabling deephot's capabilities. Their unique properties directly influence the effectiveness of thermal management strategies. The selection and integration of these materials are paramount in achieving deep penetration and intense heat management.
- Thermal Conductivity Optimization
Materials with exceptionally high thermal conductivity are essential components. These materials facilitate rapid heat transfer, minimizing thermal resistance. Examples include certain types of graphene and carbon nanotubes. In the context of deephot, these materials are strategically incorporated into designs to ensure efficient heat dissipation or absorption from microelectronics or other high-heat-generating components. Improved thermal conductivity translates into enhanced system performance by reducing overheating.
- Specific Heat Capacity Manipulation
Materials with tailored specific heat capacities can effectively absorb or release heat. A high specific heat capacity signifies a material's ability to store thermal energy without significant temperature change. This characteristic is particularly valuable in applications demanding the precise regulation of temperature. Examples include certain ceramic composites used for thermal buffering. This ability to effectively manage heat storage is vital in deephot, allowing for more stable and controlled temperature profiles.
- Material Anisotropy for Directed Heat Flow
Some advanced materials exhibit anisotropic properties, meaning their thermal conductivity varies across different directions. This characteristic allows for directional heat flow control. Using these materials strategically in deephot facilitates targeted heat dissipation, enabling precise temperature control in specific areas of a device or system. Examples include certain oriented polymer composites, where varying thermal properties in different directions influence the path of heat transfer.
- Phase Change Materials for Enhanced Heat Absorption/Dissipation
Phase change materials can absorb or release significant amounts of heat during transitions between different phases. This characteristic enables the development of highly efficient cooling or heating systems within the framework of deephot. These materials provide a powerful method for regulating temperature, leading to greater system stability and efficiency. Examples include certain metal alloys, salts, and eutectic mixtures that undergo reversible phase transitions near operational temperatures.
In summary, the precise selection and strategic integration of advanced materials are integral to the effectiveness of deephot. The superior thermal conductivity, controlled heat capacity, directional heat flow manipulation, and heat absorption/dissipation properties offered by these materials contribute to achieving deep penetration and intense heat management, leading to significant improvements in device performance and stability. The exploration and development of novel advanced materials will undoubtedly continue to enhance the scope and reach of deephot technology in diverse applications.
3. High Efficiency
High efficiency is a cornerstone of deephot. Optimized thermal management strategies are directly linked to enhanced system performance and reduced energy consumption. The pursuit of maximizing efficiency in thermal processes forms a fundamental aspect of this specialized approach. This exploration examines key facets of high efficiency within the context of deephot, demonstrating its importance and real-world applications.
- Reduced Energy Consumption
Deephot's focus on minimizing thermal resistance and maximizing heat transfer directly leads to reduced energy consumption. By optimizing heat flow, systems require less energy to maintain desired operating temperatures. This is particularly critical in applications like data centers, where energy costs are significant. Deephot's approach allows for substantial reductions in energy waste, which translates to both economic and environmental benefits.
- Improved System Performance
High efficiency in thermal management directly translates to improved system performance. By preventing overheating and maintaining optimal temperatures, deephot enables components to operate at their peak capabilities. This leads to increased processing speed, higher data throughput, and extended device lifespans in applications like microelectronics. Avoiding thermal throttling is a direct outcome of high efficiency in deephot.
- Enhanced Reliability and Durability
Consistent high performance and reduced operating temperatures translate to enhanced reliability and durability. Minimizing thermal stress on components reduces the risk of failure and extends the lifespan of devices significantly. In applications like high-performance computing and industrial machinery, the potential for long-term operational stability and reduced maintenance requirements is crucial, directly linked to the high efficiency of deephot thermal management.
- Minimized Thermal Stress
Deephot's optimized strategies to manage and dissipate heat lead to significantly minimized thermal stress on various components. This reduction in stress directly impacts longevity and reliable operation. By controlling the temperature gradients within a system, deephot reduces the likelihood of component damage and degradation resulting from overheating, significantly extending operational lifecycles in a wide range of applications.
These facets illustrate the critical role of high efficiency in deephot's overall effectiveness. Optimized thermal management through deephot approaches not only saves energy but also enhances performance and stability, making it a vital consideration for a wide range of technological applications. Further exploration of specific materials and methodologies employed by deephot will underscore the importance of high efficiency in realizing its full potential.
4. Precise Control
Precise control is a defining characteristic of deephot, underpinning its efficacy in diverse applications. Deephot's ability to meticulously manage thermal fluxes relies on precise control at both the microscopic and macroscopic levels. This control allows for targeted heat dissipation or absorption, minimizing thermal resistance and maximizing efficiency. Without precise control, the potential benefits of deephot are significantly diminished, as thermal management becomes erratic and less predictable.
Consider the application in high-performance microelectronics. Precise control of heat flow within integrated circuits is essential for preventing overheating, which can lead to performance degradation or failure. Deephot facilitates this by meticulously targeting and manipulating thermal pathways, ensuring optimal heat transfer and minimizing localized temperature spikes. Similarly, in industrial processes demanding precise temperature regulation, precise control within deephot allows for the creation of highly consistent and predictable thermal environments, leading to improved product quality and process efficiency. The ability to modulate heat transfer precisely allows for enhanced control over the temperature profile within a system, minimizing undesired thermal stresses.
The significance of precise control extends beyond specific applications. It highlights the fundamental importance of understanding and manipulating thermal dynamics at various scales. Achieving deephot's potential necessitates a meticulous understanding of the interplay between material properties, system design, and thermal behavior. Challenges in achieving precise control often stem from the complexity of the systems being managed, the inherent variability of materials, and the intricate interactions within thermal environments. Overcoming these challenges will be crucial for broader adoption and expansion of deephot's application in diverse sectors. Precise control, in essence, is not merely a component but the very essence of deephot's effectiveness and transformative potential.
5. Microscopic scale
Understanding thermal phenomena at the microscopic level is critical for the effectiveness of deephot. This involves analyzing how materials behave at the atomic and molecular scale, influencing heat transfer. This perspective is essential for designing materials and structures that optimize heat flow and minimize thermal resistance, a key driver of deephot's efficacy.
- Material Properties and Heat Transfer
At the microscopic level, the arrangement of atoms and molecules, along with their bonding and interactions, directly impacts a material's thermal conductivity. Materials with well-defined atomic structures conducive to efficient heat transport are critical in deephot applications. Certain nanomaterials, like carbon nanotubes and graphene, exhibit extraordinary thermal conductivity at this scale, making them promising candidates for optimizing heat dissipation in advanced systems. The ability to precisely control the nanoscale structure of materials allows engineers to tailor thermal properties for improved system performance.
- Interface Effects and Thermal Resistance
The interfaces between different materials often present significant thermal resistance. At the microscopic scale, these interfaces reveal areas of reduced thermal conductivity due to atomic-level irregularities or mismatches in bonding. Deephot's success relies on minimizing these interfaces or optimizing them for maximum heat transfer. This often involves utilizing specific surface treatments or specialized material configurations to minimize interfacial thermal resistance. Understanding the nanoscale characteristics of these interfaces provides strategies for designing interfaces that facilitate effective heat flow.
- Phonon Transport and Scattering
Phonons, quantized lattice vibrations, are the primary carriers of heat at the microscopic scale. The interaction of phonons with atoms and defects in the material structure influences the rate of heat transfer. Controlling phonon scattering through careful material design is essential to enhance thermal conductivity in deephot applications. Specifically, deephot aims to engineer material structures that promote phonon transport in desired directions, minimizing scattering that impedes heat flow.
- Molecular Dynamics Simulation
Microscopic simulations, such as molecular dynamics, provide valuable insights into heat transfer mechanisms. These simulations can model the behavior of atoms and molecules under various thermal conditions, helping predict and optimize the performance of materials. This level of detail is crucial in deephot, allowing engineers to test and refine material designs before extensive experimentation, ensuring more efficient and targeted applications of deephot concepts.
In essence, the microscopic scale is not merely a supporting element in deephot; it's fundamental. Understanding the intricate details of atomic and molecular interactions provides the foundation for optimizing materials and systems for heat management. Deephot's ability to effectively manage thermal fluxes hinges on the careful consideration of these microscopic interactions, paving the way for more efficient and powerful technological applications.
6. Macroscopic Scale
The macroscopic scale in deephot encompasses the overall design and operation of systems, considering the interplay of components at a larger, more readily observable level. It complements the microscopic analysis by providing a holistic view of heat flow and management within the entire system. This approach is crucial for understanding and optimizing the performance of devices and processes where high thermal efficiency is paramount.
- System Design and Configuration
Macroscopic analysis dictates the overall arrangement and layout of components. Factors like heat source location, heat sink placement, and material choices for connecting elements are all determined at this level. This configuration fundamentally influences the path and rate of heat flow throughout the entire system. Effective macroscopic design is key to minimizing thermal resistance and maximizing heat transfer, crucial components of deephot's effectiveness.
- Heat Transfer Mechanisms
At the macroscopic scale, thermal transport phenomena such as conduction, convection, and radiation are considered in their totality within the system. The interplay of these mechanisms within the defined geometry dictates the overall heat transfer rate. An accurate understanding of these interactions, including system geometry and fluid flow paths (if applicable), is essential for optimal design using deephot principles. This holistic approach to heat transfer mechanisms is crucial for accurate prediction and optimization.
- System-Level Analysis and Optimization
Macroscopic examination enables system-level analysis and optimization to identify bottlenecks and improve overall thermal performance. This analysis involves considering the interplay of different components and identifying points of significant thermal resistance. By analyzing the system holistically, deephot strategies can be optimized for maximum efficiency in managing heat flow throughout the entire structure, allowing for precise thermal management at a larger scale.
- Integration with External Environments
Considering the external environment is vital. Factors such as ambient temperature, airflow, and radiation significantly influence the thermal behavior of the system. Proper macroscopic analysis enables the design of systems that effectively mitigate the impact of external thermal conditions, preserving the system's optimal performance, even under varying external thermal profiles. Deephot strategies address these environmental influences to ensure consistently high performance and reliability.
In conclusion, the macroscopic perspective is indispensable in deephot. By combining microscopic insights with macroscopic analysis, a complete understanding of heat flow and management within a system emerges. This integrated approach enables the development of highly efficient and reliable systems across various applications, from microelectronics to industrial processes.
7. Heat Dissipation
Heat dissipation is a fundamental component of deephot. The efficient removal of heat is critical for maintaining optimal performance and preventing damage to sensitive components within various systems. Deephot strategies explicitly target enhanced heat dissipation, striving for rapid and controlled removal of thermal energy. The efficiency of heat dissipation directly influences device lifespan, operational stability, and overall performance. For example, in high-performance computing systems, the rapid dissipation of heat from processors prevents overheating, maintaining system speed and reliability. Conversely, inadequate heat dissipation can lead to system throttling, reduced performance, and potential component failure. Effective heat dissipation strategies, central to deephot, are vital for ensuring systems function optimally and extend component lifespans.
Practical applications emphasize the significance of heat dissipation. In microelectronics, efficient heat removal is essential for preventing the degradation of integrated circuits. In data centers, the effective dissipation of heat from servers is crucial for maintaining high operational capacity and minimizing energy consumption. Advanced materials, crucial in deephot, are engineered to enhance heat transfer rates. Specific designs of cooling systems, including sophisticated thermal interface materials and optimized airflow patterns, are designed to accelerate heat removal. Deephot strategies thus optimize heat dissipation pathways, minimizing resistance and maximizing thermal efficiency in diverse environments. Real-world applications showcase the direct correlation between enhanced heat dissipation and improved system performance and operational reliability. Understanding the complexities of heat dissipation and implementing strategies to improve it is paramount in achieving the goals of deephot.
In summary, heat dissipation is not just a secondary aspect; it's a defining factor in deephot's effectiveness. Effective heat removal strategies directly contribute to the performance, stability, and longevity of various systems. Optimized pathways for heat dissipation are central to deephot's approach. By understanding the intricate connection between heat dissipation and deephot's strategies, engineers can develop advanced cooling systems that drive innovation in various fields and promote more efficient use of technology. The principles and techniques employed in deephot, emphasizing efficient heat dissipation, are essential for achieving greater system performance and longevity. Furthermore, understanding these principles allows for more targeted design of thermal management solutions across diverse applications.
8. Performance Enhancement
Performance enhancement is intrinsically linked to deephot. Effective thermal management, a core aspect of deephot, directly translates to improved system performance. By minimizing thermal resistance and maximizing heat transfer, deephot enables components to operate at optimal temperatures, preventing throttling and enhancing overall functionality. This direct correlation between controlled thermal environments and system capabilities is crucial for applications demanding high performance, such as microelectronics and high-performance computing. The efficiency gains from deephot strategies, in terms of performance, are often significant and directly impact the practical utility of the system.
Real-world examples illustrate this connection. Modern microprocessors generate substantial heat, and without effective cooling mechanisms (a key element in deephot), performance would significantly degrade. Data centers rely on sophisticated cooling systems to maintain optimal server temperatures. Optimized thermal management in these scenarios, through deephot principles, directly contributes to higher throughput, reduced latency, and improved overall system efficiency. Similarly, deephot strategies are crucial for the development of portable devices, like smartphones or laptops. The ability to maintain performance under demanding workloads in portable devices is largely reliant on effective heat management, as exemplified in deephot's principles. A controlled thermal environment enables sustained operation at peak performance in a compact and portable device.
In essence, performance enhancement is not a separate entity but an integral outcome of deephot's approach. Understanding the direct causal link between optimized thermal management and performance improvements is paramount for developers and engineers working across various technological domains. By prioritizing thermal efficiency through deephot strategies, system designers can achieve significant performance boosts and enable the creation of more capable, reliable, and efficient technological solutions. The ability to maintain optimal temperatures, a core feature of deephot, directly contributes to consistent performance, a key factor in the utility of modern devices and systems.
Deephot FAQs
This section addresses common inquiries regarding deephot, a specialized thermal management approach. The following questions and answers provide clarity on key aspects and applications of this technology.
Question 1: What is deephot, and how does it differ from conventional thermal management?
Deephot represents a specialized approach to thermal management, emphasizing deep penetration and intense heat management. It leverages advanced materials and methodologies to achieve extremely high rates of heat dissipation or absorption. Conventional methods often rely on broader, less precise strategies. Deephot's distinction lies in its microscopic and macroscopic control over thermal fluxes, enabling superior performance and efficiency in managing high heat loads, often seen in advanced microelectronics and high-power systems.
Question 2: What types of materials are central to deephot's operation?
Deephot often employs materials with exceptional thermal conductivity, like certain graphene forms and carbon nanotubes. These advanced materials allow for rapid heat transfer, minimizing thermal resistance. Materials with tailored specific heat capacities also play a role, enabling precise heat storage and release. These materials, when combined with innovative design, are critical for effectively managing high heat generation rates and maintaining consistent operating temperatures.
Question 3: How does deephot enhance performance in high-power devices?
By reducing thermal resistance and enhancing heat transfer, deephot allows components to operate at optimal temperatures, preventing throttling and performance degradation. Consistent high temperatures are crucial to prevent critical damage to the components. This translates into increased processing speeds, higher data throughput, and extended component lifespans in high-power devices. Deephot's design principles are essential to achieving high performance in high-power contexts.
Question 4: What are the potential applications of deephot in various sectors?
Deephot holds potential for a wide range of applications, including advanced microelectronics, high-performance computing, data centers, and industrial processes. In each case, the need to control high heat fluxes is paramount. Deephot's optimization of thermal management enhances the reliability and efficiency of these systems.
Question 5: What are the primary challenges in implementing deephot technologies?
Implementing deephot technologies presents challenges related to material selection, design complexity, and precise control of thermal fluxes at multiple scales. Optimizing material properties for high thermal conductivity and developing intricate cooling systems are demanding tasks. A deep understanding of microscopic and macroscopic heat transfer mechanisms is essential for effective implementation.
These FAQs provide a foundational overview of deephot. Further investigation into specific applications and technical details will offer a more comprehensive perspective. The focus on precise thermal control and high-performance materials is central to the future development of deephot technologies.
Moving forward, we'll explore the specific design considerations and technological advancements driving this innovative thermal management approach.
Conclusion
This exploration of deephot has highlighted its multifaceted nature as a specialized thermal management approach. Key aspects, including precise control at microscopic and macroscopic scales, the utilization of advanced materials for enhanced heat transfer, and the resulting performance gains, have been meticulously examined. The analysis underscores the critical role of deep penetration and intense heat management in achieving optimal system functionality and longevity. The importance of heat dissipation strategies and their direct impact on performance have been emphasized, demonstrating deephot's suitability for a broad range of applications. The interplay between material properties and system design, central to deephot's effectiveness, has been demonstrated, showcasing the complex interplay of factors in achieving high thermal efficiency.
Deephot represents a significant advancement in thermal management, promising substantial improvements in performance, reliability, and energy efficiency across various technological sectors. The future of deephot hinges on continued research and development in advanced materials and precise control mechanisms. This exploration serves as a foundation for further investigations, driving innovation in areas requiring extreme thermal control and high performance. A deeper understanding of the microscopic underpinnings and the development of more sophisticated macroscopic implementation strategies will be key to unlocking the full potential of deephot technology. The potential impact of deephot on fields requiring intensive heat management is substantial, warranting continued attention and exploration.
You Might Also Like
Nina Aouilk Parents: Who Are They?David And Rebecca Muir Wedding: A Celebration Of Love!
Taylor Mathis Fap
DeeDee Autopsy: Uncover The Truth
Pioneer Woman's Death Shocks Fans - Obituary Details Emerge
Article Recommendations
- Pat Sajak The Wheel Of Fortune Host
- My Skarsgrd Latest News Updates
- Steve Harvey Diddy A Surprising Connection


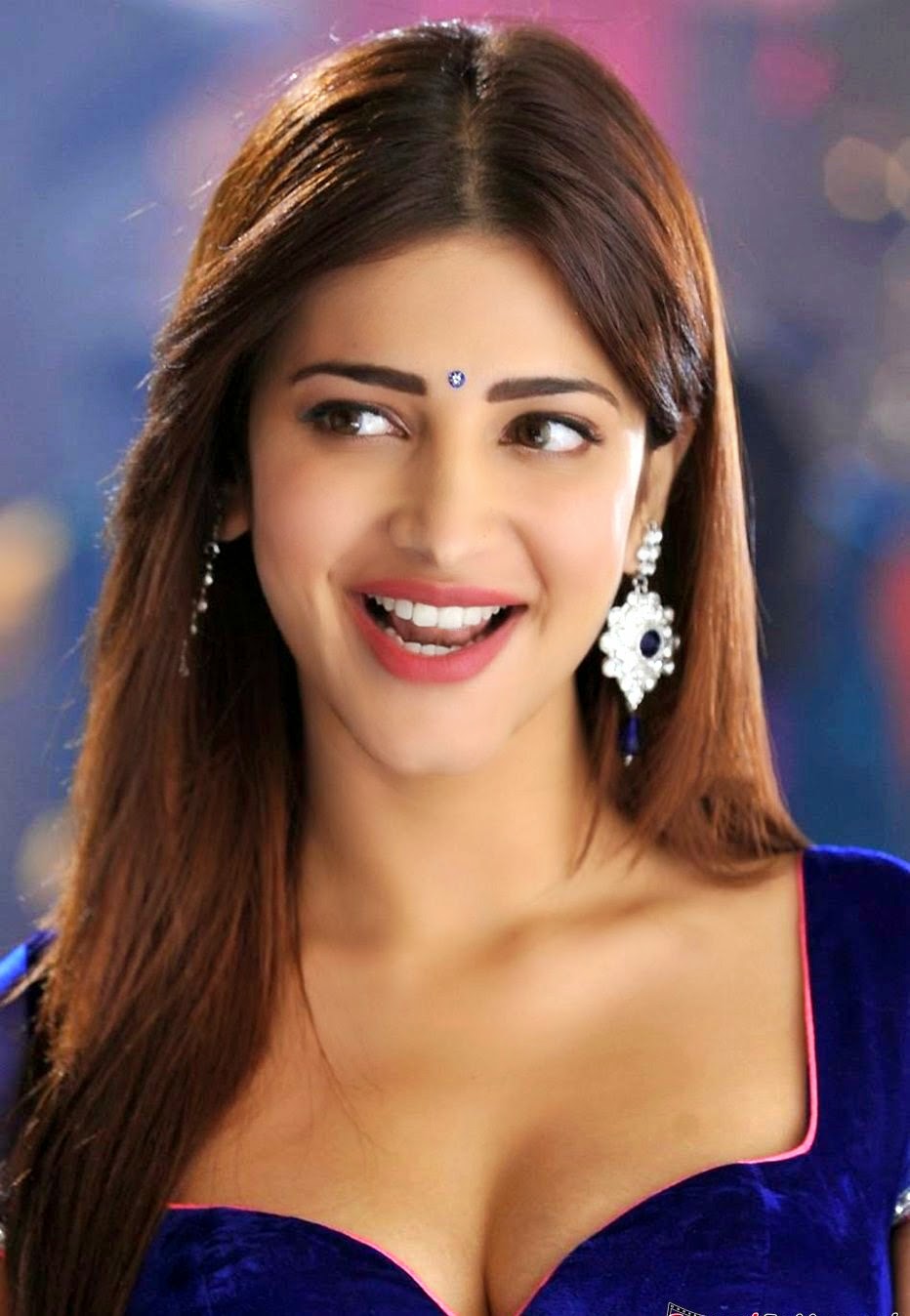